Quantum computing has the potential to tackle some of our most pressing global issues, from climate change to food security. We’re dedicated to building a full-scale, fault-tolerant quantum computer that can help solve these challenges, and I’m frequently asked where quantum computing will have its biggest impact. The answers are coming more clearly into focus, and include, most notably, the simulation of chemical interactions and materials on the quantum level. Reaching practical quantum advantage will require progressing across three quantum computing implementation levels; here, I want to connect these topics to the field of quantum networking, to bring further clarity on how our industry as a whole will progress.
As with quantum computers, quantum networks are not meant to replace their classical counterparts. In fact, classical networking will remain the foundation of this technology. Quantum networking will extend the existing networks to enable the exchange of quantum information—whether between quantum computers or classical endpoints. In turn, this means that a quantum network has the potential to unlock new capabilities by connecting remote quantum computers, solving larger-scale problems distributed on quantum clusters, and enabling precision metrology through entangled sensor networks.
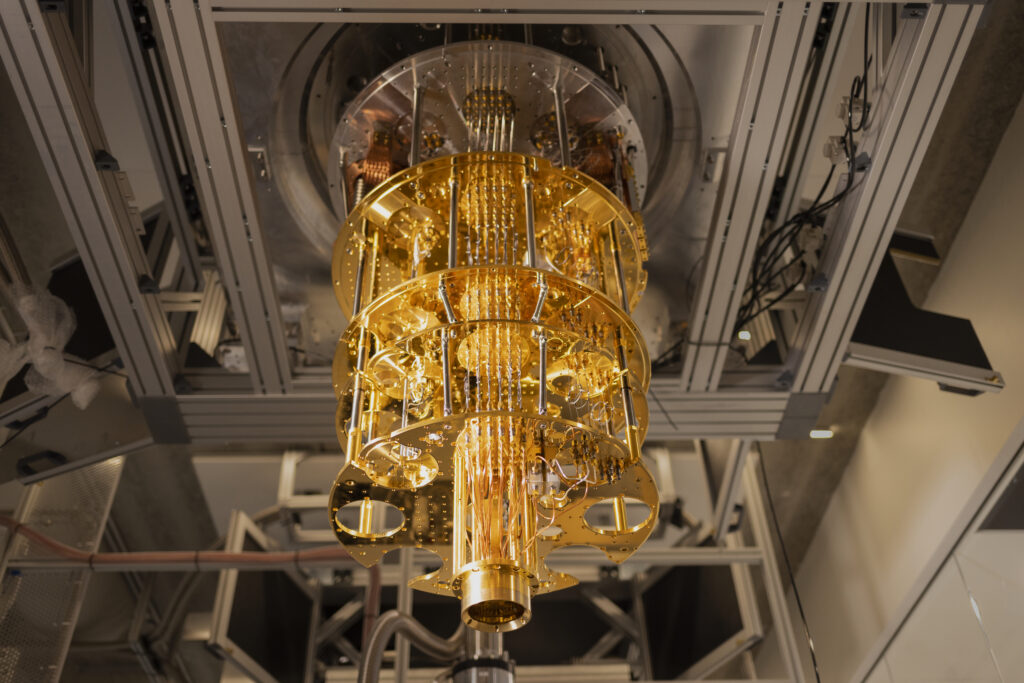
A new networking paradigm
At its core, quantum communication concerns the sending and receiving of quantum information. Whereas today’s conventional communication systems are based on classical physics, quantum communication employs the principles of quantum mechanics. The key to quantum networking is the sharing of quantum entanglement. For instance, to transmit (or “teleport”) a qubit state in a quantum network, both the sender and receiver first share a generic resource: two entangled qubits—each getting one of the two. When the sender is ready to transmit a particular qubit state, they entangle it with their half of the entangled pair and measure. This produces two bits to send to the receiver over a classical network, who uses these and the other half of the entangled pair to reconstruct the self-same state.
With this in mind, we are looking for an intentional approach to defining quantum network challenges that captures interoperability across each layer of a “quantum networking stack.” I am currently thinking about this as evolving through three stages.
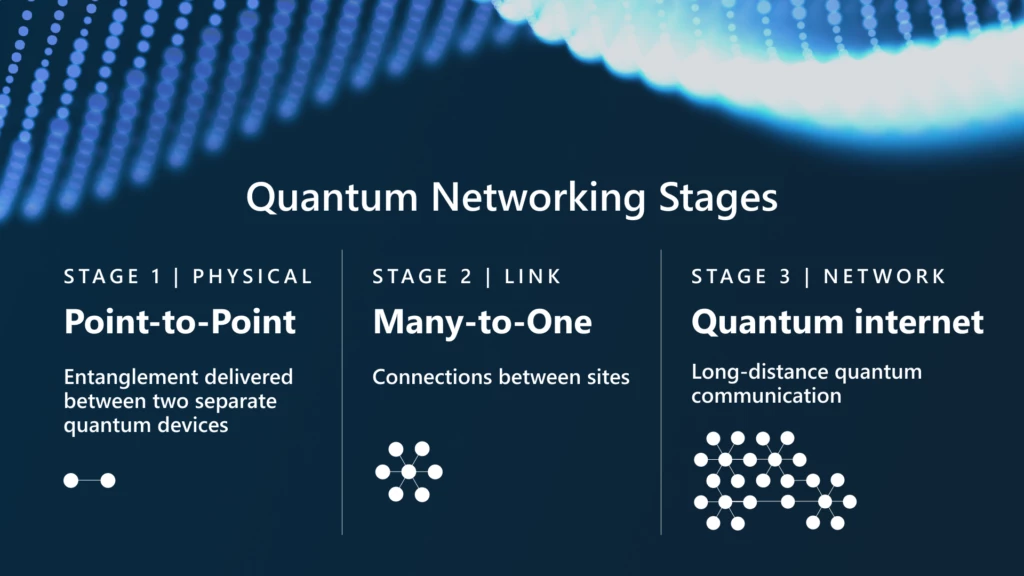
- Stage 1: Any network is built on top of point-to-point connections. I expect that the initial stage of quantum network development will be defined by technology that enables a quantum analog of Physical layer of the networking stack, where entanglement can be established between two separate quantum devices.
- Stage 2: As there are limitations to scaling point-to-point connections, I view the next stage of quantum networking as being defined by technology that enables the analog of a Link layer. At this stage, a quantum device can support and manage connections with many sites, delivering entanglement to any two as required.
- Stage 3: The final stage of development should be characterized by technology that enables a Network layer for reliable long-distance quantum communication through a complex network, which relies on resilient quantum hardware at the sites.
I recognize that the mapping of the technological stages to networking layers is not perfect. Notably, a critical device to overcome distance limitations in a quantum network will be the quantum repeater. Such a device will perform entanglement swapping to reliably extend the distance between which two devices can become entangled, and so belongs to Stage 3 technologies. Yet in the networking stack, it is part of the Physical layer. Nonetheless, I feel that the driving factor in the future development of quantum networks will revolve around network connectivity, enabled by continuously improving quantum hardware.
Enabling a quantum internet
I imagine that “the quantum internet” can mean very different things to different people. Perhaps it is best then to discuss a quantum internet, which simply refers to a large system of distributed quantum computers interconnected with quantum links. This quantum internet is a separate, but co-existent, network alongside a classical network, which, in fact, might be the internet.
Today, there are several approaches for establishing entanglement between nearby noisy quantum machines (NISQ) in labs, so currently, we are in the first stage towards developing a quantum internet. However, to scale truly large networks, we believe it’s important to build upon current technology and use photons at telecom wavelengths.
It’s impossible to scale a network where each pair of sites must communicate through a point-to-point connection. Thus, the second stage in this roadmap is to develop quantum devices that can on-demand distribute entanglement to multiple quantum endpoints. For instance, a “quantum hub” would have as its sole purpose distributing entanglement to any two neighboring sites, thereby relieving them of the need to have point-to-point connections with each other. Such a device could then enable a quantum local-area NISQ network.
One might view such a quantum hub as a NISQ repeater, as from the endpoint’s perspective their communication has made one “hop” through the network. However, without resilient quantum hardware one cannot expect useful entanglement to survive more than a handful of such hops, restricting the network to a local area.
In this language, a quantum internet may be considered as a wide-area quantum network, which requires establishing entanglement between distant endpoints through multiple hops through the network. In the third stage of quantum networking, we can accomplish these hops through a method called “entanglement swapping” and ensure reliability by employing methods such as entanglement distillation.
Quantum key distribution
The envisioned stages of quantum networking are from the perspective of transmitting quantum information between remote quantum devices. However, these stages may also be appropriate for quantum networks between classical sites. This is the case for quantum key distribution (QKD), where the only requirement at the endpoints is to create or detect photons. Current QKD hardware, based on the “BB84” protocol, may be considered in stage one as it relies on a point-to-point connection between two QKD devices. In the QKD protocol “E91”, a central device distributes entangled pairs to the end-users, and so a QKD system that uses this protocol could be considered as stage two. Device-independent QKD additionally performs self-testing to ensure the correct behavior of the system; while an imperfect analogy, this could be considered akin to reliability and so form stage three of development.
Today, QKD is considered part of the quantum-safe effort to provide security systems that are not vulnerable to quantum cryptanalysis. Although it does provide a different approach to some cryptographic tasks, it has fundamental technical limitations and therefore cannot be viewed as a complete solution. At Microsoft, our Quantum Safe migration effort is focused on post-quantum cryptographic algorithms, as recommended by cybersecurity agencies globally. Read more about our efforts in the space.
Application of quantum networks
As per the title of this blog, I have focused on quantum networking from the view of creating a quantum internet. Having separated endpoints is foundational for many quantum communication applications. For example, the security of some quantum protocols for anonymous voting relies on the voters being separated. In quantum metrology one uses the phenomenon that measuring half of an entangled system instantaneously affects the other half, regardless of the distance between them, to enable precise timing and position verification. In distributed quantum computing, blind computing protocols allow one party to delegate the computation of a quantum algorithm to another without revealing the input, output, or even the algorithm that was run.
Nonetheless, one should not think the only value of quantum networking is linking distant quantum computers. Modern supercomputers are built from many networked computing nodes that can operate as a single system. Perhaps future quantum computers will follow a similar design; the stages of development above would apply equally to switching and routing of quantum information in such a quantum cluster.
Next steps with Azure Quantum
There is still lots of work ahead, and as an industry we must continue to separate signal from noise when evaluating technological progress. However, as I continue to engage with both customers and our Azure Quantum team, my excitement for the possibilities ahead of us only grows. I believe that the collective genius and input from the community are important for refining the framing of quantum networking. We invite your comments and perspectives so that we can make progress together toward its future. For more information, you can visit the following resources:
- You can visit our latest blog about the Quantum Computing Implementation Levels and recent achievements in this space.
- You can learn more about how we’re accelerating scientific discovery with supercomputing capabilities, AI, and quantum computing with Azure Quantum Elements.
- You can discover more about our comprehensive approach to quantum safety.